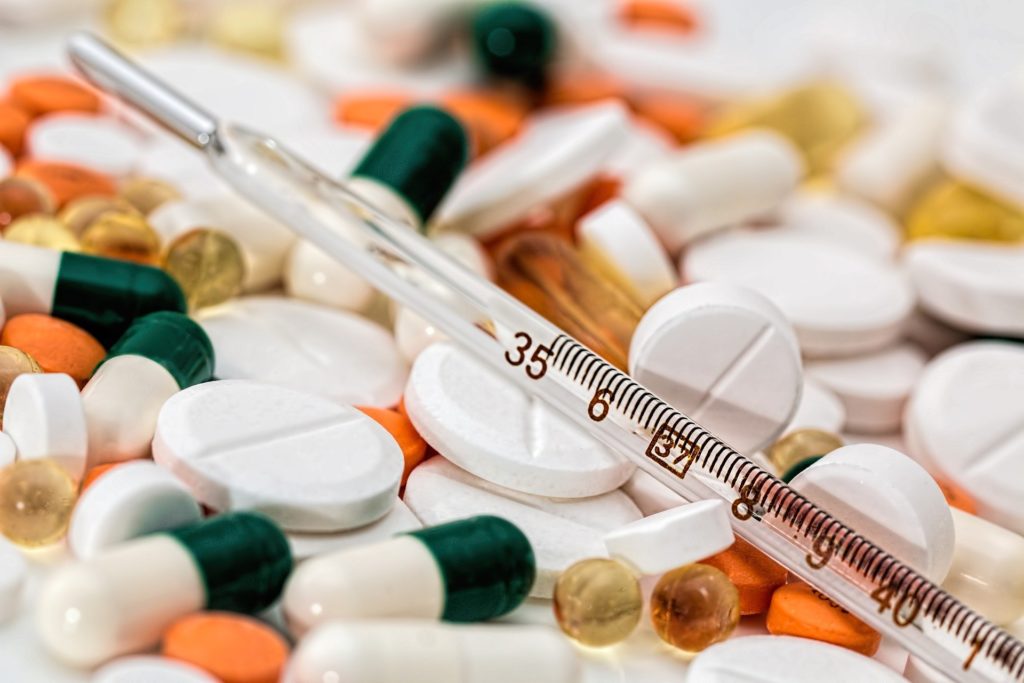
Groundbreaking research presented at UEG Week 2024 reveals a promising new treatment for type 2 diabetes (T2D) that could significantly reduce or eliminate the need for insulin therapy.
This innovative approach combines a novel procedure called ReCET (Re-Cellularization via Electroporation Therapy) with semaglutide, resulting in the elimination of insulin therapy for 86% of patients.
Globally, type 2 diabetes (T2D) affects 422 million people, and obesity is recognized as a significant risk factor. While insulin therapy is commonly used to manage blood sugar levels in T2D patients, it can lead to side effects such as weight gain and further complicate diabetes management.
In the initial human trial, 14 participants between the ages of 28 and 75, with body mass indices ranging from 24 to 40 kg/m², underwent the ReCET procedure while under deep sedation. This treatment aims to enhance the body’s responsiveness to its own insulin. After the procedure, the participants followed a two-week isocaloric liquid diet, and then the dosage of semaglutide was gradually increased to 1mg per week.
At the 6- and 12-month follow-up, 86% of participants (12 out of 14) no longer needed insulin therapy, and this positive outcome persisted through the 24-month follow-up. During this time, all patients were able to maintain glycemic control, with HbA1c levels staying below 7.5%.
The maximum dose of semaglutide was well-tolerated by 93% of participants, one individual could not increase to the maximum dose due to nausea. All patients successfully completed the ReCET procedure, and no serious adverse effects were reported.
Dr Celine Busch, lead author of the study, commented, “These findings are very encouraging, suggesting that ReCET is a safe and feasible procedure that, when combined with semaglutide, can effectively eliminate the need for insulin therapy.”
“Unlike drug therapy, which requires daily medication adherence, ReCET is compliance-free, addressing the critical issue of ongoing patient adherence in the management of T2D. In addition, the treatment is disease-modifying: it improves the patient’s sensitivity to their own (endogenous) insulin, tackling the root cause of the disease, as opposed to currently available drug therapies, that are at best disease-controlling.”
Looking ahead, the researchers plan to conduct larger randomised controlled trials to further validate these findings. Dr Busch added, “We are currently conducting the EMINENT-2 trial with the same inclusion and exclusion criteria and administration of semaglutide, but with either a sham procedure or ReCET. This study will also include mechanistic assessments to evaluate the underlying mechanism of ReCET.”