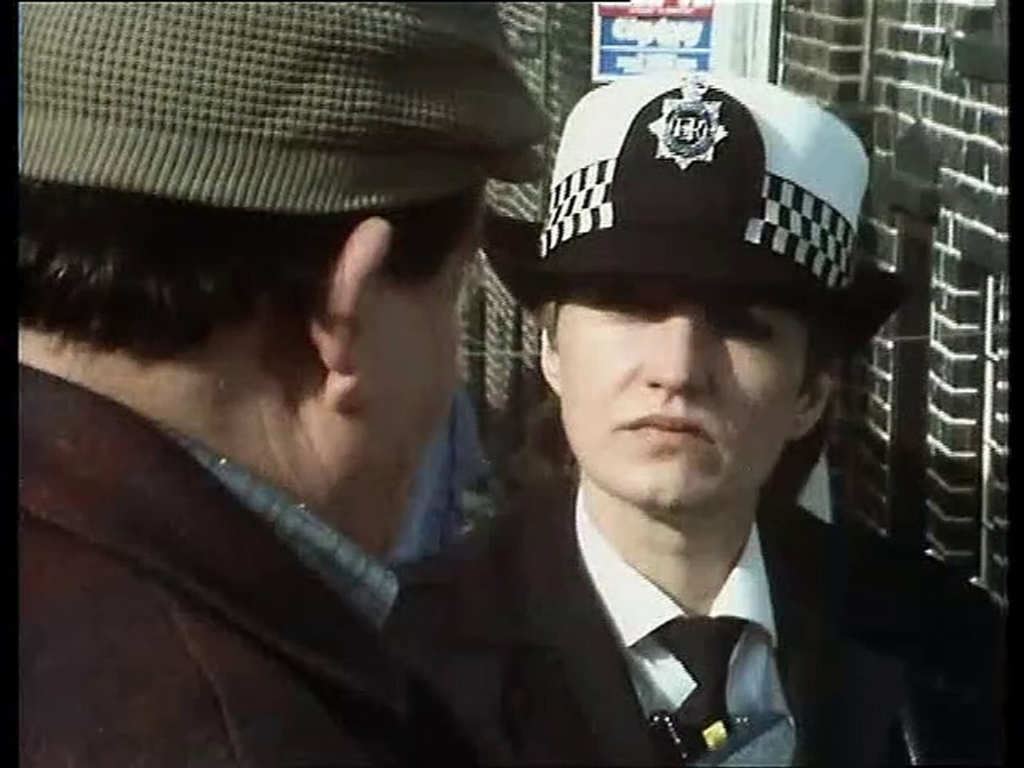
Researchers at Flinders University tested the belief that autistic adults are more likely than non-autistic adults to be criminally exploited due to difficulties in recognizing criminal intent.
“It is not uncommon for defence lawyers, often with the backing of testimony from ‘expert’ witnesses, to claim that autistic adults struggle to interpret the intentions of others or understand their thoughts. This difficulty can make them more susceptible to being lured into criminal activity,” says Professor Neil Brewer, Matthew Flinders Distinguished Emeritus Professor of Psychology in the College of Education, Psychology, and Social Work.
“Such arguments reflect the widely-held perspective that difficulties reading others’ intentions, emotions, and motivations are fundamental features of autism.
However, this perspective may not withstand scrutiny, and we found that, in general, autistic adults are no more vulnerable to being involved in criminal acts than non-autistic adults.
“Furthermore, the difficulties in mindreading often associated with autism are not universally present among autistic adults.”
In a study published in the American Psychological Society’s journal, Law and Human Behavior, former PhD student Zoe Michael and her supervisor, Professor Neil Brewer, developed a new and realistic approach called the Suspicious Activity Paradigm (SAP). This paradigm was designed to evaluate how effectively adults can recognize and respond to cues that indicate social interactions may lead to criminal behaviour.
The study included 197 participants: 102 autistic adults and 95 non-autistic adults, who role-played in scenarios that progressively indicated criminal intent from their interactions.
They were asked about their reactions at different stages as the scenarios developed to evaluate their ability to recognize and respond to suspicious actions from others, thus gauging their susceptibility to being unknowingly drawn into criminal activities.
“We found that, overall, both autistic and non-autistic adults responded in similar ways to suspicious behaviour across various scenarios,” says Professor Brewer.
“Importantly, autistic adults did not show lower rates of suspicion or adaptive responses when compared to their non-autistic counterparts as the scenarios unfolded. Nor did they take longer to recognise the potentially problematic nature of the interaction.”
Building on previous research, the study found that verbal intelligence and Theory of Mind (ToM) – a term used to describe the ability to take the perspective or read the mind of others – predicted someone’s ability to recognise and respond to suspicious activity.
“Our findings indicate that the ability to understand others’ perspectives and intentions – and not the presence of an autism diagnosis – was a critical factor influencing their vulnerability to crime,” he says.
In other words, while autistic individuals who had difficulty discerning others’ intentions were vulnerable, the same was true of their non-autistic peers.
It is important to note, however, that a relatively small proportion of autistic individuals’ performance on the mindreading measure was below that of any of the non-autistic sample, a finding consistently replicated by the Flinders research team that developed the measure.
This indicates that there will be some autistic individuals who will likely be particularly vulnerable because of mindreading difficulties – but such challenges cannot be assumed.
“Thus, rather than defence lawyers and clinicians assuming, and arguing, that a diagnosis of autism automatically signals a particular vulnerability to being lured into crime, it is important to formally assess and demonstrate that a criminal suspect or defendant has significant mindreading difficulties that likely have rendered them vulnerable,” he adds.